The atmosphere consists of many different particles in suspension (aerosols) that vary in size distribution and chemical composition based on the region of the atmosphere, the anthropogenic activity, and meteorological conditions. With a dimension range from a few nanometers to tens of micrometers and atmospheric lifetime as long as several weeks, these particles can affect the regional as well as the global climates.
Gaining insights into the chemical composition of aerosols is essential because of their potential adverse effects on human health and environment. To assess the impact, it is necessary to understand the physico-chemical composition of aerosols at the level of the single particle. However, physico-chemical characterization of aerosols at the micron scale is a challenging task because of the small size of typical aerosol particles.
Analytical Techniques
The electron beam method such as scanning electron microscopy coupled to energy dispersive X-ray microanalysis (SEM-EDX) are generally utilized to perform micrometer spatial resolution characterization of the elemental composition and internal structure of particles. The ion beam techniques such as time of flight secondary ionization mass spectrometry (ToF-SIMS) can provide the composition of the very first nanometers of the surface. Nevertheless, neither ToF-SIMS nor SEM-EDX can acquire data from the sample under ambient conditions as they require low pressure operation, which may change the structure of aerosol particles.
In comparison,Confocal Raman microspectrometrythat integrates the spatial resolution of optical microscopy and the molecular analysis capabilities of Raman scattering can acquire molecular information from individual micron size aerosol particles at ambient conditions. The availability of automated Raman systems enables acquisition of 2D molecular images with a lateral resolution limited by light diffraction. Nonetheless, the broad chemical heterogeneity of aerosol particles, often coupled with a large luminescence background, can cause adverse overlapping of spectral data and can affect the widespread adoption of Raman microscopy in the analysis of the internal chemical structure of aerosol particles.
自动映射和相关的结合interactive self-modeling mixture analysis (Multivariate Curve Resolution) to treat Raman images can provide key insights into the molecular characteristics and distribution of molecular species within samples. This method is very useful in the sample mixture analysis where the spectroscopy and chemistry are vague. A set of spectral data for a mixture of different components with varying composition is enough to provide the results.
This curve resolution technique can help identifying the number of constituents in a mixture and extracting the spectra of each component, including fluorescence signal and respective semi-quantitative concentration profiles. Using the extracted spectra, each component is identified through library searches. Spatially resolved chemical data about the heterogeneity and speciation of environmental samples can be acquired from the reconstructed molecular images that are generated from the resolved spectra and their respective contributions.
Characterizing Particles Emitted from Smelters
Smelters emit micron and sub-micron sized particles into the atmosphere. Depending on weather conditions, these particles can travel long distances. Raman images of airborne particles are depicted in Figure 1, showing aggregation of windblown lead (Pb) containing particles along with other atmospheric particles released by smelters. Cascade impaction was used for particle collection. The Raman spectra for PbSO4(anglesite), (NH4)2SO4, and PbSO4.PbO (lanarkite) were decoupled utilizing the technique described above, in spite of their spectral similarity. However, the smoothing procedure of the Raman imaging software was not used.
Figure 1.Raman imaging of lead containing particles emitted in the atmosphere by lead smelter facility. (a) optical image; (b) spectra of the 80 pixels of the image; Resolved Raman spectra of (c) PbSO4; (d) PbO.PbSO4; (e) (NH4)2SO4; Raman images of (f) PbSO4; (g) PbO.PbSO4; (h) (NH4)2SO4.
Airborne Contamination of Plants
Industrial airborne pollution results in the deposition of lead-containing particles at the surface of leaves of terrestrial plants. These particles then penetrate into the leaf by crossing the leaf cuticle. Understanding lead speciation within the leaves is helpful in assessing risk of population exposure to atmospheric metal contamination.Raman microimagingutilizing UV laser excitation (266nm) was employed to determine spatially-resolved lead speciation in lettuce exposed to atmospheric Pb smelter-originated fallouts for 43 days.
The leaves must be probed with UV excitation because of the emission of strong fluorescence by biological molecules such as chlorophyll when excited with visible radiation. Hence, in the spectral region of 400-800nm, the fluorescence signal overlaps and masks any underlying Raman features. Since the fluorescence and Raman spectra are amply isolated in the UV region, they do not interfere with each other.
.jpg)
Figure 2.Tentative pathways for lead uptake after deposition of Pb-containing particles. Deposition of particles on the leaf surface (1), chemical transformation on the leaf surface leading to secondary Pb-containing phases and possibly solutes (2). Toxicity symptoms (necroses) induced by the presence of the contaminated particles on the leaf (3).
The identification of lead compounds deriving from primary particles beneath the cuticle of the contaminated leaves and the toxicity symptoms induced by the compounds in the form of necrotic zones (necroses) are demonstrated in Figure 2. Optical images, Raman spectra and molecular images of the lead-rich necrotic zone on the leaf surface are shown in Figure 3.
.jpg)
Figure 3.Optical images, Raman spectra and molecular images of the lead-rich necrotic zone on the leaf surface.
Conclusion
The results clearly demonstrate the value ofhigh spatial resolution Raman confocal microscopyin the field of environmental analysis. The technique can also be applied in the analysis of heterogeneous gas-aerosol particle reactions, which are critical in atmospheric chemistry.
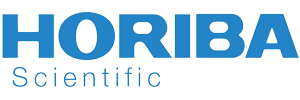
This information has been sourced, reviewed and adapted from materials provided by HORIBA Scientific.
For more information on this source, please visitHORIBA Scientific.